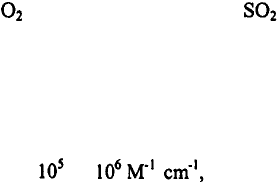
BIOLOGICAL APPLICATIONS OF QUANTUM DOTS
41
model coincides with the structure of qdots, in which mobile carriers (the particle) are
confined within the dimensions of the qdot (the box) with discrete emission wavelengths
(wavefunctions) and bandgap energy levels. As the physical dimensions of the box
become smaller, the bandgap energy increases.
The fluorescence emission of qdots is extremely stable upon photoexcitation. In
comparative measurements of qdots with small organic fluorophores, qdots have been
shown to be ~ 100 times more stable against photobleaching. Qdots have also been
shown to be more photostable than fluorescent proteins (e.g., phycoerytherin), although
this result has not been quantified.
18
The photobleaching of qdots is believed to arise
from a slow process of photo-induced chemical decomposition. This hypothesis is
supported by our observation of a shift in emission-colour from red to blue when qdot-
aggregates are spread on a glass slide and monitored under an epifluorescence
microscope with high-power UV-excitation. Henglein and coworkers speculated that
CdS decomposition is initiated by the formation of S or SH radicals upon optical
excitation.
19
These radicals can react with from the air to form an complex,
resulting in slow particle degradation. Capping the surface of qdots with a thick second
semiconductor layer has produced qdots with excellent photostability.
Due to the bright luminescence and high photostability of qdots, single qdots can be
easily visualized and imaged under a conventional epifluorescence microscope. In
addition, qdots are brighter than most small organic dye molecules due to their large
molar extinction coefficients. Bawendi and coworkers
20
estimated that the molar
extinction coefficients of CdSe qdots are about to depending on the
particle size and the excitation wavelength. These values are 10-100 times larger than
those of organic dyes, but are similar to the absorption cross sections of phycoerytherin, a
multi-chromophore fluorescent protein. It has been estimated that a single qdot is
approximately equivalent in fluorescence intensity to 10 to 20 small organic dye
molecules.
21
In this section, we have briefly described some of the properties of qdots that make
them appealing for biological applications. For a more in-depth look at the physical
chemistry of qdots, the interested reader can refer to a number of excellent reviews by
Alivisatos
22
, and Murray et al.
23
SYNTHESIS AND CHARACTERIZATION OF QUANTUM DOTS
3.
The most commonly synthesized semiconductor nanocrystals are composed of atoms
from groups II-VI (e.g. CdSe‚ CdTe‚ CdS‚ and ZnSe) and groups III-V (e.g. InP and
InAs) of the periodic table.
24-29
In particular‚ rapid advancements in synthesis and
characterization techniques have led to the development of highly luminescent and
monodisperse CdSe qdots.
16‚30-32
In the following sections‚ we will discuss a number of
different approaches to the synthesis and characterization of high-quality qdots.
Synthesis Techniques
3.1.
Typical techniques for the synthesis of semiconductor qdots involve the growth of
nanocrystals using molecular precursors in either aqueous or organic solutions. In one
approach to qdot synthesis in aqueous media‚ solutions of cadmium and sulphur
precursors are injected into hot aqueous solutions containing stabilizing agents or in