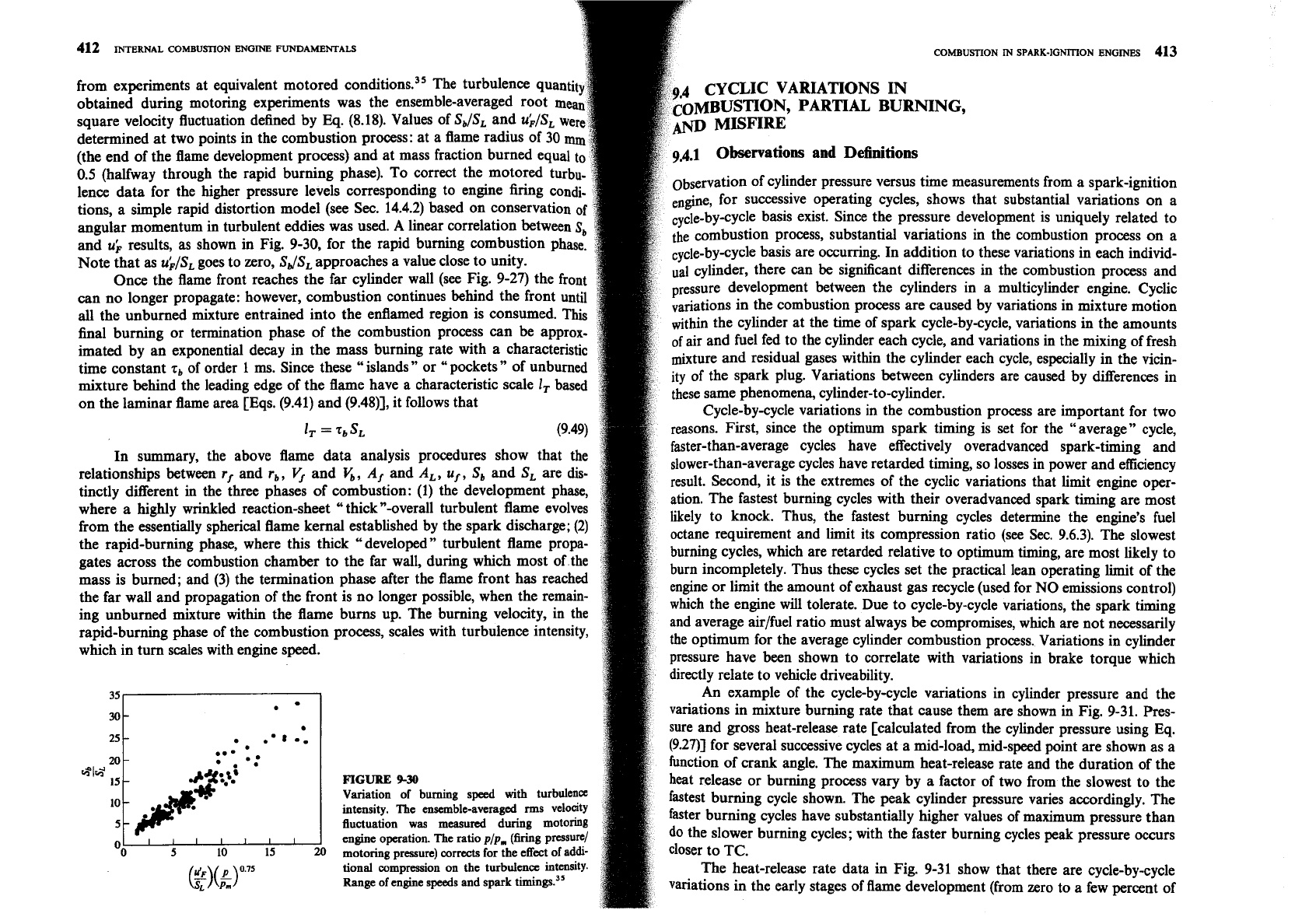
412
INTERNAL COMBUSTION ENGINE FUNDAMENTALS
COMBUS~ON
IN
SPARK-IG~N
ENGMES
413
from experiments at equivalent motored
condition^.^^
The turbulence q
ARIATIONS
IN
obtained during motoring experiments was the ensemble-averaged root
,
PARTIAL BURNING,
square velocity fluctuation defined by
Eq.
(8.18). Values of Sb/SL and ~$3,
determined at two points in the combustion process: at a flame radius of
(the end of the flame development process) and at mass fraction burned eq
9.4.1
Observations and Definitions
0.5 (halfway through the rapid burning phase). To correct the motored tu
observation of cylinder pressure versus time measurements from a spark-ignition
lence data for the higher pressure levels corresponding to engine firing
engine, for successive operating cycles, shows that substantial variations on a
tions, a simple rapid distortion model (see Sec. 14.4.2) based on conservati
cycle-by-cy~le basis exist. Since the pressure development is uniquely related to
angular momentum in turbulent eddies was used.
A
linear correlation between
S,
the combustion process, substantial variations in the combustion process on a
and uk results, as shown in Fig. 9-30, for the rapid burning combustion phase.
cycle-by-cycle basis are occurring. In addition to these variations in each individ-
Note that as u;/SL goes to zero, SJSL approaches a value close to unity.
ual cylinder, there can be significant differences in the combustion process and
Once the flame front reaches the far cylinder wall (see Fig. 9-27) the front
pressure development between the cylinders in a multicylinder engine. Cyclic
can no longer propagate: however, combustion continues behind the front until
variations in the combustion process are caused by variations in mixture motion
all the unburned mixture entrained into the enflamed region is consumed.
This
within the cylinder at the time of spark cycle-by-cycle, variations
in
the amounts
final burning or termination phase of the combustion process can be approx-
of
air and fuel fed to the cylinder each cycle, and variations in the mixing of fresh
imated by an exponential decay in the mass burning rate with a characteristic
mixture and residual gases within the cylinder each cycle, especially in the
vicin-
time constant z, of order 1
ms.
Since these "islands" or "pockets" of unburned
ity of the spark plug. Variations between cylinders are caused by differences in
mixture behind the leading edge of the flame have a characteristic scale
I,
based
these same phenomena, cylinder-to-cylinder.
on the laminar flame area [Eqs. (9.41) and (9.48)], it follows that
Cycle-by-cycle variations in the combustion process are important for two
I,
=
rb SL
reasons. First, since the optimum spark timing is set for the "average" cycle,
faster-than-average cycles have effectively overadvanced spark-timing and
In summary, the above flame data analysis procedures show that the
slower-than-average cycles have retarded timing, so losses in power and e6ciency
relationships between rj and r,,
V/
and
6,
Aj and A,, u,, S, and SL are dis-
result. Second, it is the extremes of the cyclic variations that limit engine oper-
tinctly different in the three phases of combustion: (1) the development phase,
ation. The fastest burning cycles with their overadvanced spark timing are most
where a highly wrinkled reaction-sheet
"
thickv-overall turbulent flame evolves
likely to knock. Thus, the fastest burning cycles determine the engine's fuel
from the essentially spherical flame kernal established by the spark discharge; (2)
octane requirement and limit its compression ratio (see Sec. 9.6.3). The slowest
the rapid-burning phase, where this thick "developed" turbulent flame propa-
burning cycles, which are retarded relative to optimum timing, are most likely to
gates across the combustion chamber to the far wall, during which most of-the
burn incompletely. Thus these cycles set the practical lean operating limit of the
mass is burned; and (3) the termination phase after the flame front has reached
engine or limit the amount of exhaust gas recycle (used for
NO
emissions control)
the far wall and propagation of the front is no longer possible, when the remain-
which the engine will tolerate. Due to cycle-by-cycle variations, the spark timing
ing unburned mixture within the flame bums up. The burning velocity, in the
and average
air/fuel ratio must always be compromises, which are not necessarily
rapid-burning phase of the combustion process, scales with turbulence intensity,
the optimum for the average cylinder combustion process. Variations in cylinder
which in turn scales with engine speed.
pressure have been shown to correlate with variations in brake torque which
directly relate to vehicle driveability.
An example of the cycle-by-cycle variations in cylinder pressure and the
variations in mixture burning rate that cause them are shown in Fig. 9-31. Pres-
sure and gross heat-release rate [calculated from the cylinder pressure using Eq.
(9.2711 for several successive cycles at a mid-load, mid-speed point are shown as a
function of crank angle. The maximum heat-release rate and the duration of the
FIGURE 9-30
heat release or burning process vary by a factor of two from the slowest to the
Variation of burning speed
with
turbulena
fastest burning cycle shown. The peak cylinder pressure varies accordingly. The
intensity. The ensemble-averaged
rms
velocity
fluctuation
was
measured during motoring
faster burning cycles have substantially higher values of maximum pressure than
engine operation. The ratio
p/p,
(firing pressure1
do the slower burning cycles; with the faster burning cycles peak pressure occurs
35
30-
25
20
@I*
15
lo:
5
0~1'11'1'
5
10
15
20
motoring pressure) corrects for the eff'ect of addi-
tional compression on the turbulence intensity.
($)@
0'15
The heat-release rate data in Fig. 9-31 show that there are cycle-by-cycle
Range of engine speeds and spark
timing.35
variations in the early stages of flame development (from zero to a few percent of
.
-
..I
*.
.
..
.
.
*:
d*::!
-