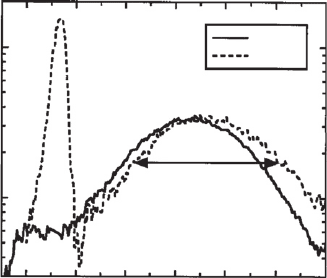
Optical and Vibrational Properties of Self-assembled GaN Quantum Dots 249
7.4.1 Confi nement effects
Increased thermal stability and improved emission effi ciency at room temperature are well-
established consequences of zero-dimensional confi nement and common probes for identifying
QD luminescence. In GaN/AlN heterostructures the 2.8 eV band gap mismatch between the dot
and the barrier materials ensures a deep localization potential. Indeed, strong carrier confi ne-
ment was already demonstrated in the fi rst optical studies performed on GaN/AlN QDs grown in
the SK mode by MBE [23] and by MOCVD [17, 84] and on GaN/Al
x
Ga
1
x
N ( x 0.2) QDs grown
in the VW mode by using Si as a surfactant [16, 85] . Figure 7.17 shows the resonantly excited
PL spectrum of a GaN/AlN QD superlattice grown by MBE on a commercial GaN substrate at two
different temperatures. While the emission from the bulk GaN substrate is visible at low tempera-
ture only, a broader emission band centred at 3.75 eV and attributed to the GaN QDs remains
almost unchanged, both in shape and intensity, with temperature. The blue shift of the QD emis-
sion with respect to the bulk band gap is a consequence of the confi nement potential and the
large inhomogeneous broadening reveals the wide distribution of dot sizes probed in this experi-
ment. The integrated intensity of the QD emission stays constant up to room temperature, as can
be seen in Fig. 7.18 , because migration to non-radiative centres is hindered by strong confi ne-
ment. On the contrary, a severe quenching in the PL intensity of GaN bulk and QW systems is
observed as temperature increases due to the high density of threading dislocations and other
defects that act as non-radiative traps.
Bulk GaN
GaN quantum dots
T 300K
T 2K
3.4 3.5 3.6 3.7 3.8 3.9 4
Photon energy (eV)
PL intensity (arb. units)
Figure 7.17 PL of a 20 period GaN/AlN QD superlattice grown by MBE on a GaN MOCVD-grown substrate.
The excitation source was the 302 nm (4.1 eV) line of an argon laser which lies below the WL emission energy at
4.7 eV [23] . With permission from AIP© 1998.
In the same way as in other self-assembled systems, GaN QDs also present a large inhomogene-
ity in QD sizes resulting in large inhomogeneous broadening of the emission spectrum, see Fig.
7.17 . A possible strategy for reducing the dispersion in island size is the growth of vertically cor-
related QD multilayers. As already described in section 7.2, when increasing the number of layers
the vertical self-alignment is accompanied by the homogenization of the QD size distribution in
successive dot planes and an average increase of their diameter [37, 38] . The spacer layer acts like
a bandpass fi lter that prevents the formation of QDs of smaller size. Figure 7.19 shows the room
temperature PL spectra of samples containing 1, 3, 5, and 10 layers of buried QDs grown by
MOCVD [38] . Besides the emission from the QDs, a higher energy peak centred at 4.7 eV is attrib-
uted to the emission of the WL. In order to evidence the effects of the stacking in the multilay-
ered samples, the PL spectrum of the reference single layer can be subtracted, see the inset of Fig.
7.19 , and it is shown that the PL from the upper layers is red shifted in energy and shows a clear
reduction of the spectral linewidth when compared to that of the single-layer sample. Another
interesting observation is that the intensity of the emission increases with the number of layers
CH007-I046325.indd 249CH007-I046325.indd 249 6/27/2008 5:16:04 PM6/27/2008 5:16:04 PM